Research background
“If a craftsman wants to do good work, he must first sharpen his tools.”
― Confucius, The Analects
Scientific instruments are vital for humanity to understand the world and explore the unknown. As Mendeleev stated, " Science begins where one begins to measure," and instrument innovation is a cornerstone of scientific breakthroughs. It's also a key area for China to become a global scientific and technological powerhouse and develop new productive forces.
Technologies like X-ray diffraction (Nobel Prize in Physics, 1914 and 1915), super-resolution fluorescence microscopy (Nobel Prize in Chemistry, 2014), and cryo-electron microscopy (Nobel Prize in Chemistry, 2017) allow us to study the structure and function of various proteins and organelles within individual cells with unprecedented precision. This has greatly advanced life sciences and medicine. However, these techniques can only cover a small field of view, making it difficult to dynamically observe a large number of cells in a living environment, much like "looking at a leopard through a tube."
CT imaging (Nobel Prize in Physiology or Medicine, 1979) and magnetic resonance imaging (Nobel Prize in Physiology or Medicine, 2003) can provide a large imaging field of view, enabling the study of the heterogeneity of different organs at the macroscopic scale in living organisms. These techniques have been widely used in clinical medicine, making significant contributions to human health. However, their imaging resolution is far from reaching the single-cell level.
Cells are the fundamental units of life. At every moment, a symphony of interactions between different types of cells is taking place within the human body. However, there is a significant technological gap at the mesoscale, which connects the microscopic and macroscopic worlds. This makes it challenging for current research to simultaneously observe the spatiotemporal heterogeneity of a large number of cells in different physiological and pathological states at the organ scale in living mammals. This greatly limits the development of fields such as neuroscience, immunology, oncology, and pharmacology.
In neuroscience, for example, the interaction and function of a large number of neurons give rise to functions such as intelligence and consciousness. Understanding the structure and activity patterns of neural circuits is essential for unraveling the workings of the brain. However, traditional microscopes with single-neuron identification capabilities typically have only a millimeter-level field of view, allowing them to cover only a single or a few brain regions in mice and record neural signals dynamically on a single plane. Functional MRI, while capable of three-dimensional whole-brain observation, lacks the spatial resolution to identify individual cells. Consequently, the U.S. BRAIN Initiative, launched in 2013, listed "large-scale neural network recording technology" as one of its nine major funding areas.
Similarly, in oncology, only by combining a large field of view with high resolution can we comprehensively capture the entire process of tumorigenesis and development. This enables more precise research into different drug responses and the discovery of new drug targets.
Ten years it takes to grind a sword, Its frosty edge has yet to be tested.
Targeting this international frontier challenge, Professor Qionghai Dai 's team at Tsinghua University received support from the National Natural Science Foundation of China's Major Scientific Instruments Development Project as early as 2013. They pioneered research in the field of mesoscale in vivo microscopic imaging and successfully developed the world's first gigapixel mesoscale fluorescence microscope, RUSH, in 2018. RUSH is capable of simultaneously achieving centimeter-level field of view and subcellular resolution.
Although RUSH was hailed by international peers as a pioneer in mesoscale microscopic imaging, its complexity and high cost limited its use to only a few scientists at the time. Moreover, the RUSH system still faced a series of bottlenecks, including: how to achieve high-speed three-dimensional imaging using a two-dimensional sensor; how to avoid cell damage (i.e., phototoxicity) caused by prolonged laser exposure, enabling long-term, high-speed observation; how to overcome optical aberrations and background interference caused by complex imaging environments; how to improve signal-to-noise ratio under low-light conditions; and how to efficiently process large-scale mesoscale data. Each of these technical bottlenecks is an international challenge in the field of biomedical imaging. Simultaneously addressing these in vivo imaging barriers within a single system is even more daunting.
"The world's wonders and extraordinary sights often lie in remote and perilous places, rarely reached by humans. Thus, only those with determination can reach them." This is an ancient poem by Anshi Wang from Song dynasty. In the following six years, Professor Qionghai Dai 's Imaging and Intelligent Technology Laboratory, aiming for the peak of in vivo mesoscale microscopic imaging, persevered and continuously tackled these international frontier challenges. They successively proposed key theories and technologies such as scanning light field imaging principles, digital adaptive optics architecture, virtual scanning algorithms, confocal scanning light field architecture, and self-supervised denoising algorithms. These achievements were published in prestigious international journals like Cell, Nature, Nature Biotechnology, and Nature Methods, addressing a series of barriers in mesoscale in vivo microscopic imaging and laying the foundation for a new generation of mesoscale in vivo microscopes.
Because all these technological developments shared a common goal—to achieve truly practical in vivo mesoscale microscopic observation—they seamlessly integrated within the same system architecture, leading to a revolutionary improvement in overall performance.
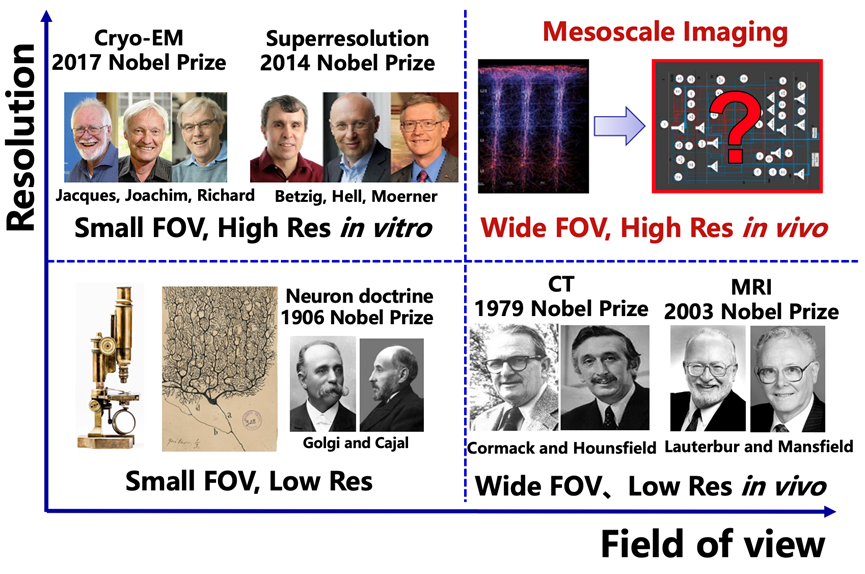
Figure 1. The missing gap of mesoscale imaging
Building The Pillars of a Great Power
On September 13, 2024, a new study titled "Long-term mesoscale imaging of 3D intercellular dynamics across a mammalian organ" was published in Cell, with Qionghai Dai, Zengcai Guo, and Jiamin Wu from Tsinghua University as co-corresponding authors. The study announced the advent of the next-generation mesoscale in vivo microscope, the RUSH3D system. This remarkable instrument not only boasts a centimeter-level three-dimensional field of view and subcellular resolution but also achieves continuous low-phototoxicity observation for up to tens of hours at a high-speed three-dimensional imaging rate of 20Hz. Compared to the most advanced commercial spinning disk confocal microscopes currently available, RUSH3D offers a nearly hundredfold increase in imaging field area at the same resolution, a tenfold increase in three-dimensional imaging speed, and a hundredfold reduction in phototoxicity (resulting in a hundredfold increase in effective observation duration). This unprecedented multi-scale imaging capability across space and time provides a fresh perspective for studying complex biological processes. With this system, researchers can, for the first time, dynamically observe tissue heterogeneity at cellular resolution on the mammalian organ scale in a panoramic manner, opening the door to studying the interactive behavior of large-scale, diverse cells in vivo.
The interdisciplinary research team utilized RUSH3D to achieve high-speed three-dimensional observation covering layers 2/3 of the cerebral cortex in live mice at single-cell resolution. They captured the response patterns of various cortical regions under multi-sensory stimulation and tracked large-scale neural responses with single-neuron precision for several consecutive days. Additionally, they observed the immune response in multiple brain regions after acute brain injury, revealing the migration and reflux of numerous neutrophils from non-vascular areas into the brain. For the first time, they simultaneously observed the formation of multiple germinal centers within lymph nodes and the migration of T cells between different germinal centers during the immune response in mice. These preliminary experiments merely scratch the surface of RUSH3D's potential applications, showcasing its vast prospects for cutting-edge research in neuroscience, immunology, oncology, and pharmacology.
The development and commercialization of this instrument (by Zhejiang Hehu Technology Co., Ltd.) fills the gap in mesoscale in vivo observation of complex life phenomena. It advances novel computational imaging theories and key technologies, significantly enhancing China's research and application capabilities in high-end scientific instruments. RUSH3D provides a new "killer app" for uncovering new phenomena and mechanisms in neuroscience, oncology, and immunology, enabling Chinese life scientists and medical researchers to take the lead in addressing major fundamental research questions using domestically developed high-end instruments.
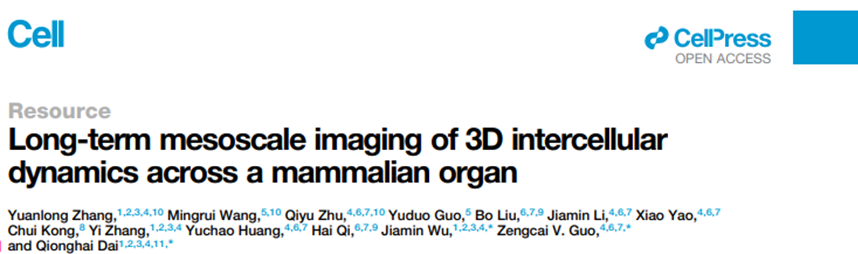
Computational imaging is a novel concept that overturns the age-old notion of "what you see is what you get." By utilizing multi-dimensional computational methods such as computational coding and computational acquisition, computational imaging has evolved from the "what you see is what you get" of human vision to the "what you compute is what you get" of machine vision. This has led to a series of new features that surpass the physical limitations of acquisition hardware, including ultra-fast frame rates, ultra-high resolution, and ultra-high signal-to-noise ratio.
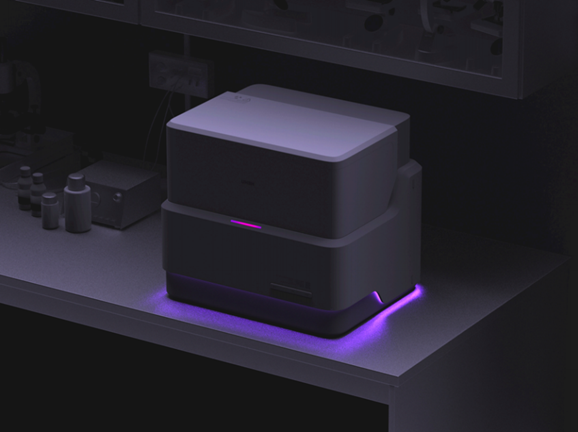
Figure 2. RUSH3D system overview
The RUSH3D system integrates a series of imaging and intelligent technology theories and key technologies previously developed by the team. Based on the principle of scanning light field imaging, it can achieve high-speed three-dimensional imaging within an axial range of 400 microns while maintaining low phototoxicity. To address the issue of optical aberrations severely affecting the system's imaging resolution, RUSH3D acquires ultra-fine four-dimensional spatial angle data across the entire field of view. It constructs a wave optics-based digital adaptive optics architecture (wDAO) that does not require additional wavefront sensors or spatial light modulators in the optical system. This allows for large field-of-view, multi-region adaptive optics aberration correction at the backend, thereby improving the spatial resolution and signal-to-noise ratio of three-dimensional imaging in complex environments. This design enables effective overcoming of spatially non-uniform system aberrations and sample aberrations using only conventional-sized objective lenses, achieving uniform high spatial resolution gigapixel imaging across the entire field of view. This significantly reduces the size and cost of mesoscale imaging systems, making high-resolution in vivo mesoscale microscopic imaging systems more widely accessible.
Furthermore, in in vivo fluorescence imaging, background fluorescence severely affects the signal-to-background ratio. RUSH3D employs a multi-scale background removal algorithm to separate background signals from in-focus signals, accurately filtering out out-of-focus background and thereby improving the signal-to-background ratio, recovering fluorescence signals submerged in the fluorescent background. Further integration with confocal scanning light field will enable confocal imaging fidelity while maintaining high-throughput mesoscale observation capabilities.
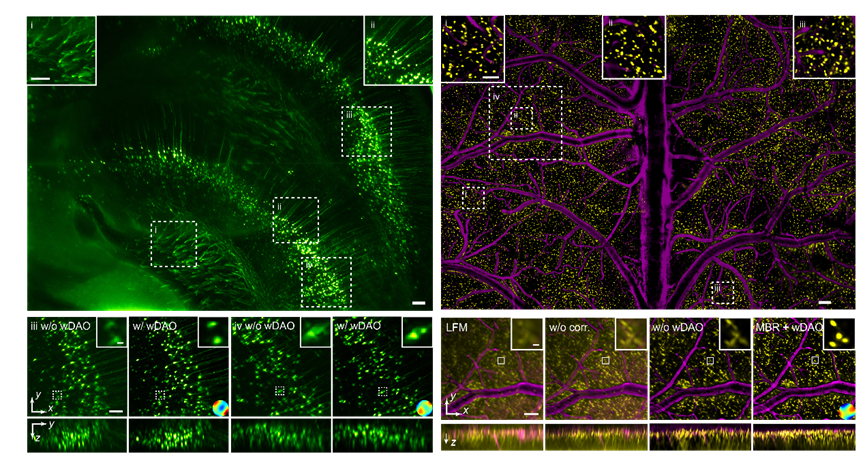
Figure 3. RUSH3D imaging results across whole field-of-view
Pioneering Imaging Technology Enables Efficient Neuroscience Research
RUSH3D, with its multi-scale imaging capabilities across space and time, significantly broadens scientists' understanding of the brain. The neuronal network in the cerebral cortex is considered a crucial and complex information processing center in the nervous system of higher animals, playing a key role in generating biological intelligence and even consciousness. Previous research has revealed that functional differentiation in the cortex leads to the formation of cortical regions that regulate various types of information. The functional activities and information exchange between neurons in different cortical regions are essential for the cortex to perform its functions. However, due to limitations in observation techniques, most current research can only simultaneously record neuronal activity in one or a few cortical regions in experimental animals, making it difficult to further study the joint dynamic changes of cortical neuronal networks. By leveraging the advantages of RUSH3D's large field of view, three-dimensional high resolution, and high frame rate, the interdisciplinary team has pioneered long-term recording of near 100,000 neurons in 17 brain regions of the dorsal cortex in head-fixed awake mice. They can also perform multi-trial recordings of the same group of neurons across multiple days. Using this recording method, they confirmed that neurons responding to sensory stimuli and regulating movement are not limited to a single sensory cortex or motor cortex but are widely distributed across various cortical regions. However, the ability of neurons in different regions to encode, integrate, and distinguish sensory information varies. Furthermore, they discovered a caudal-to-rostral propagation pattern in the mouse cortical neuronal network during the initiation of spontaneous motor behavior. This result suggests that the integration of information from visual, tactile, and other sensory cortical neurons and the diffusion of signals across the entire cortex may be key factors in triggering spontaneous movement. Building on this, RUSH3D is expected to enable, for the first time, the analysis of three-dimensional neural functional connectivity across the entire dorsal cortex. This involves functional clustering of three-dimensional neuronal populations based on their activity under different tasks and stimuli, as captured by RUSH3D, to depict a three-dimensional functional connectivity map of millions of neurons. This will help unravel the mysteries of brain consciousness, advance research on neurodegenerative diseases, and further promote brain-inspired artificial intelligence research.
Figure 4. Cortex-wide neuronal imaging
Breaking through In Vivo Imaging Bottlenecks Drives New Discoveries in Immunology
The RUSH3D system provides an unprecedented tool for studying large-scale cell interactions. For example, germinal centers (GCs) are structures formed by the aggregation of antigen-activated B cells under the guidance of chemotaxis. Due to limitations in field of view and recording duration, the process of how immune cells participate in the generation of multiple GCs and migrate between them has never been continuously recorded. The team used RUSH3D to perform high-speed three-dimensional imaging of lymph nodes near the inguinal region of mice during an immune response for over ten hours, spanning approximately 85 hours. This allowed them to completely record the process of B cells participating in the generation of multiple GCs. Simultaneously, they observed the complete migration of some T cells between multiple GCs 145 hours into the immune response, after the GCs had fully formed. This suggests that T cells may be involved in information exchange and transmission between GCs. RUSH3D has achieved, for the first time, continuous in vivo observation of immune responses at the whole-organ scale, particularly the entire process from tumorigenesis to immune response and tumor growth, fully revealing the immune activation response triggered by tumors. RUSH3D's observation of messenger T cells exchanging information between different germinal centers is expected to uncover new immune mechanisms and unveil the mystery of tumor metastasis from a mesoscale in vivo perspective for the first time.
Revolutionary Cross-scale Precise Analysis for Medical Research
The multi-scale imaging capabilities across space and time of RUSH3D significantly improve experimental efficiency. For instance, drug screening experiments can achieve significantly higher efficiency in a single experiment, eliminating the need for multiple trials. Moreover, because RUSH3D can provide sufficient data for statistical analysis, cross-individual differences can be effectively mitigated in a single experiment. Additionally, rare events such as tumor metastasis will no longer be random occurrences under RUSH3D observation but rather routinely visible phenomena. With its advantages in low-phototoxicity three-dimensional observation, the system can also simultaneously observe long-distance communication between cells and, by tracking millions of cells over extended periods, reveal the physical laws of collective cell behavior and the mechanisms of functional emergence. As an initial exploration of RUSH3D in the medical field, researchers observed, for the first time, the migration of neutrophils throughout the entire cerebral cortex after acute brain injury. When a mouse's brain is impacted, a large number of neutrophils flow from the perivascular non-vascular areas into the brain parenchyma. There are also rare cases of cells flowing back from the brain parenchyma into blood vessels, suggesting that some cells may enter the brain parenchyma through the glymphatic system and flow back into blood vessels to transmit information to other parts of the body. Notably, this mesoscale in vivo long-term observation of the brain injury repair process is the first of its kind internationally. RUSH3D's facilitation of in-depth analysis of immune cells in brain injury will aid in developing specific therapies to prevent brain dysfunction caused by tissue edema after acute brain injury. It is also expected to provide new perspectives in a wide range of medical fields.
Technological Achievement Transformation, Safeguarding People's Health
Based on the core patents of this series of achievements, Tsinghua University has established a domestically owned and controlled advanced microscope company, Zhejiang Hehu Technology Co., Ltd. The company is dedicated to developing and producing high-end optical microscopes with internationally leading performance and promoting their cutting-edge applications in life sciences and other fields. It has already supported over 20 innovative life science research projects in oncology, immunology, neuroscience, and other fields at top domestic research institutions such as Tsinghua University, Peking University, Beihang University, Beijing Normal University, PLA General Hospital, and Tongji Hospital, serving the biopharmaceutical industry. One typical example is Professor Yu Li's team at Tsinghua University, who utilized this instrument to discover, for the first time in mammals, the generation process and function of a new organelle called "migrasome" in tumors and immunity, providing a new path for early tumor diagnosis and treatment. Professor Yang Changqing's team at Tongji Hospital affiliated with Tongji University used this instrument to reveal the critical role and core mechanism of Mas signaling in drug-induced liver injury, providing valuable data support for the development of new drug intervention targets. Professor Wang Wei's research group at Tongji Medical College of Huazhong University of Science and Technology is using the RUSH3D instrument to explore the neural circuit basis of acupuncture. Academician Shi Songhai, Professor Liu Jia, and other research groups at Tsinghua University are using the RUSH3D instrument to explore the biological basis of consciousness.
Yuanlong Zhang, Wang Mingrui, and Zhu Qizheng from Tsinghua University are the co-first authors of this paper. Professor Qionghai Dai, Professor Jiamin Wu, and Professor Zengcai Guo from Tsinghua University are the co-corresponding authors. Yuduo Guo, Bo Liu, Jiamin Li, Xiao Yao, Chui Kong, Yi Zhang, Yuchao Huang, and Hai Qi participated in the research and made significant contributions. This work was generously supported by the National Natural Science Foundation of China, the National Key Research and Development Program of the Ministry of Science and Technology, 1 and the Brain-X Program of Tsinghua University.